Researchers at the University of Southern California (USC) have developed a unifying approach that could inform the development of more effective techniques for the control of flapping wing micro air vehicles.
The researchers behind the study are part of the autonomous microrobotic systems laboratory, which is the inheritor of a research line that started at UC Berkeley more than 20 years ago, continued at Harvard from 2005 and arrived at USC in 2013.
“We, as a team, share the same dream of many in the microrobotics community; the vision of creating fully autonomous artificial insects that can operate intelligently in highly unstructured environments,” said Prof. Nestor O Perez-Arancibia, the principal investigator for the study.
“Personally, I am inspired by nature. Our machines, including Bee+, which is an amazing piece of engineering, still lag behind real insects in terms of aerobatic capabilities, actuation, sensing, computational power, etc.”
Perez-Arancibia has been conducting research into microrobotic systems for several years. He believes that all human-made machines could ultimately be improved if drawing inspiration from nature, for instance by observing and replicating the behaviour or characteristics of bees, butterflies and mosquitos.
In their study, Perez-Arancibia and his colleagues investigated the control of flying insects using two different experimental platforms: a Bee-like two-winged robot and the four-winged Bee+ micorobot developed at USC.
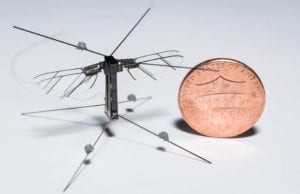
In a series of experiments, they were able to demonstrate that a technique that employs quaternion coordinates for attitude control, which is designed to control unmanned vehicles with four rotors, can be applied to drive both these robotic insects.
“We were able to simplify something that many researchers see as very difficult,” said Perez-Arancibia. “I like to believe that this is an instance in which intelligent modelling, we can understand and analyse seemingly intractable complex phenomena that can help us to develop better robotic designs, especially in terms of aerodynamic force generation, mechanism configuration and actuation.”
The results of the tests suggest that the generic strategy introduced could be used to control different types of artificial insects that share some common characteristics. While they specifically demonstrated the effectiveness of this strategy on a two-winged robot and the four-winged Bee+ robot, it could potentially also be applied to other insect-like microrobots.
Perez-Arancibia believes that by following a high-level control approach that decouples the problems of actuation and flight control, it is possible to identify general algorithms that can be employed in multiple platforms.
For instance, this approach could allow researchers to develop new algorithms for aerobatic flight employing 19-gram quadrotors that could subsequently be implemented on a 95-milligram, four-winged Bee+.
“This is relevant because as robotic flyers become smaller, they also become harder to fabricate, more difficult to handle and more expensive,” said Perez-Arancibia.
“Consequently, the fact that the same approach can be employed to synthesise controllers for platforms with weight differences as large as two orders of magnitude is significant and relevant from both research and practical perspectives.”
In the future, the approach introduced by this team of researchers at USC could inform the design and implementation of new controllers for insect-like microrobots.
In addition, over the next few months, Perez-Arancibia and his colleagues will be working on a different project aimed at creating the very first fully autonomous sub-gram flying artificial insect.
“Currently, the main limitation that has prevented microroboticists from achieving autonomous flight at the sub-gram scale is the extremely low energy densities of electric batteries,” said Perez-Arancibia. “We will achieve autonomy by employing artificial muscles powered by catalytic reactions.”